What is an SMPS?
A switched-mode power supply (SMPS) is a type of power supply used to convert electrical power efficiently from one form to another. The SMPS can convert AC (alternating current) into DC (direct current) or vice versa, and it is widely used in everything from computers, smartphones, TVs, to power adapters, and more.
The main advantage of SMPS over traditional linear power supplies is its efficiency. SMPS is capable of delivering a stable voltage and current to power electronic devices with minimal energy loss, making it ideal for applications where heat dissipation needs to be minimized.
In this article, we’ll explore how an SMPS works, its advantages, why flyback converters are commonly used, and how to design an efficient green mode SMPS for low power consumption.
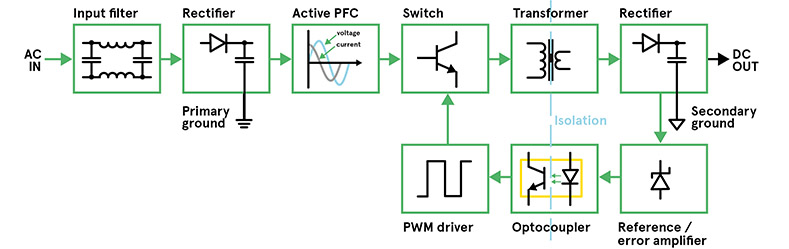
Advantages of SMPS
- High Efficiency: SMPS operates with high efficiency by minimizing energy wastage. This is mainly due to its fast switching and regulation techniques.
- Compact Size: Since SMPS doesn’t rely on large transformers, it tends to be more compact compared to traditional power supplies.
- Wide Input Voltage Range: SMPS can handle a wide range of input voltages, making it suitable for use in various regions worldwide.
- Minimal Heat Generation: With its higher efficiency, SMPS generates very little heat, making it ideal for devices where heat buildup is a concern.
Basic Working of SMPS
Let’s break down the working of an SMPS into key stages. Understanding the SMPS block diagram will help clarify its operation:
- AC Input: The input AC voltage (from the mains power supply, e.g., 110V or 230V depending on the region) is fed into the system.
- Rectification: The rectifier stage converts the AC voltage into pulsating DC using diodes.
- Filtering: A filtering stage, typically made of capacitors, smooths out the pulsating DC and removes ripple, resulting in a more stable DC voltage.
- High-Frequency Switching: The heart of an SMPS is the high-frequency switch, usually a MOSFET or power transistor. This component rapidly switches the DC on and off at a high frequency (in the kilohertz or megahertz range), converting the DC signal back into AC.
- Transformer: The high-frequency AC is then passed through a transformer. The transformer helps to step up or step down the voltage depending on the design of the SMPS.
- Output Rectification: The secondary side of the transformer generates AC, which is then rectified back to DC by diodes.
- Output Filtering: An output capacitor smooths out the DC further, ensuring a stable output.
- Control Circuit: The control circuit monitors the output voltage and adjusts the switching cycle of the MOSFET to maintain a stable output.
Why Use a Flyback Converter in SMPS?
In an SMPS design, there are several converter topologies to choose from. However, the flyback converter is the most commonly used topology for several reasons:
- Galvanic Isolation: The flyback converter provides galvanic isolation, meaning there is no direct electrical connection between the input and output, which enhances safety by protecting against voltage spikes and noise.
- Simplicity: The flyback converter has a simpler design and requires fewer components compared to other converter types, such as forward or push-pull converters. This makes it cost-effective and easy to manufacture.
- Energy Storage: The flyback converter uses a transformer to store energy when the switch (MOSFET) is on and releases the energy when the switch is off. This allows the flyback converter to handle multiple output voltages, which is beneficial in applications that require different output levels.
- Efficiency: Flyback converters achieve high efficiency, especially at low power levels, which is crucial in battery-operated devices to prolong battery life.
- Reduced Component Stress: In discontinuous conduction mode (DCM), the current in the transformer falls to zero during each switching cycle. This reduces stress on the components, making the system more reliable.
How Does a Flyback Converter Work?
Let’s brush up on the working of a flyback converter:
- DC Voltage Input: After rectification and filtering, a DC voltage is fed into the flyback converter.
- MOSFET Switching: The MOSFET (or another active switch) is rapidly switched on and off. When the MOSFET is on, current flows through the primary winding of the transformer, storing energy in its magnetic field.
- Energy Release: When the MOSFET turns off, the stored magnetic energy in the transformer’s primary winding induces a voltage in the secondary winding. This voltage forward-biases the diode on the secondary side, allowing current to flow and produce a rectified DC output.
- Output Filtering: The rectified DC is then smoothed by an output capacitor, which helps to reduce any remaining ripple.
- Feedback Control: The feedback loop monitors the output and adjusts the switching cycle to maintain a stable voltage output.
Green Mode Power Supply
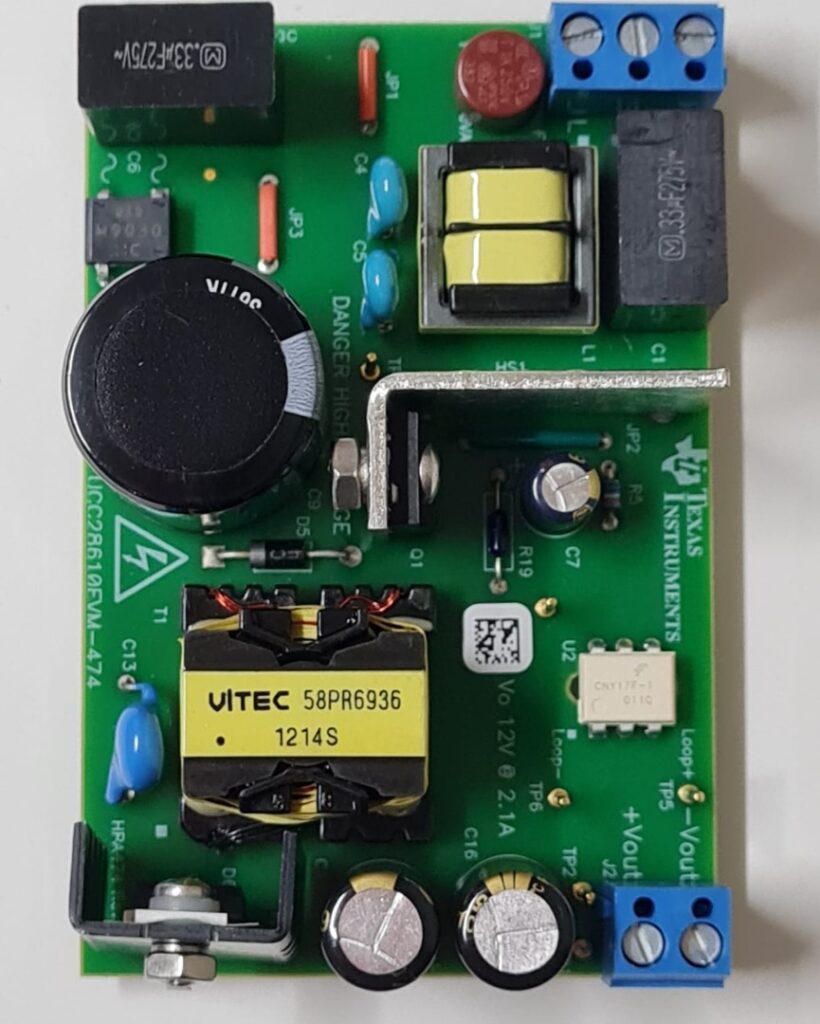
In modern power electronics, reducing energy consumption and minimizing wasted power is crucial. This is where a green mode power supply comes into play. A green mode power supply operates in such a way that when the load is low or the system is in standby mode, it consumes minimal power. This helps save energy and reduces environmental impact.
For example, in a smart TV scenario, when you pause a movie and leave the TV idle for a while, the TV will switch to standby mode. During this time, a green mode power supply ensures that the TV consumes only a fraction of the power, waiting for your next interaction.
Designing a Green Mode SMPS Using Flyback Converter
Now, let’s move on to designing a green mode SMPS. For this example, we’ll design a 25W power supply using the UCC28610 IC, which is a green mode flyback controller from Texas Instruments (TI).
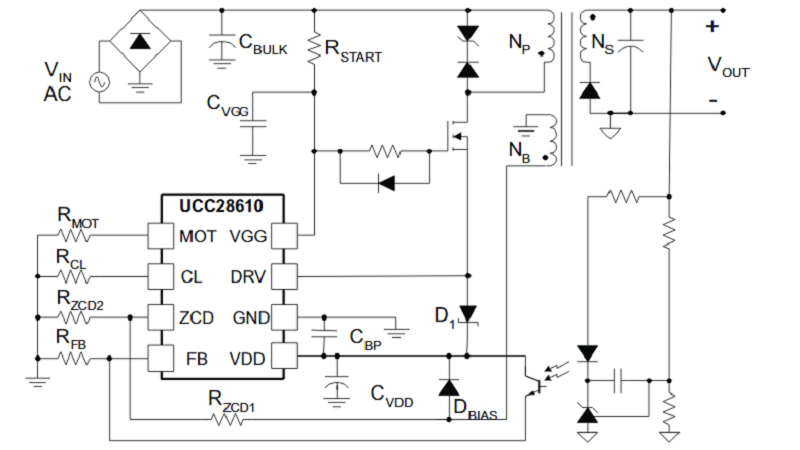
Key Features of the UCC28610 IC:
- Low No-Load Power Consumption: The UCC28610 operates with low power consumption when there is no load, making it ideal for standby applications.
- High Efficiency: The IC works in discontinuous conduction mode (DCM), reducing switching losses and improving efficiency at lighter loads.
- Cascode Driver: The IC uses a cascode driver to drive the external MOSFET, which provides fast startup and lower input power under no-load conditions.
- Current Mode Control: The feedback uses current mode control instead of voltage mode, reducing power consumption during no-load operation.
Design Steps:
- Determine the input and output specifications: Choose the input voltage range (e.g., 90V to 265V AC) and output voltage (e.g., 12V DC).
- Select the Transformer: Choose a transformer with an appropriate turn ratio to provide the desired output voltage.
- Choose the MOSFET: Select a high-voltage MOSFET that can handle the input voltage and switching frequency.
- Set Feedback and Control Circuit: Use the UCC28610’s feedback pin to regulate the output voltage by monitoring the current and adjusting the switching duty cycle.
By designing this power supply using the UCC28610 IC, we ensure low energy consumption during standby and high efficiency when the load is active.
You get guidance here to make a battery charger with cc cv function.
Learn more here to make your charger: Understanding SMPS (Switched-Mode Power Supply): How it Works and How to Design ItConclusion
SMPS is a versatile and efficient power supply solution used in a wide range of electronic devices. Among various topologies, the flyback converter is favored for its simplicity, cost-effectiveness, and ability to provide galvanic isolation. Designing a green mode SMPS using controllers like the UCC28610 ensures minimal energy waste during low-load or standby conditions, which is essential for energy-saving applications.
In future posts, we will delve deeper into the practical design steps, including schematic diagrams and component selection, for creating an efficient 25W green mode SMPS using the flyback converter topology.
Stay electrified and keep learning!
References:
- Texas Instruments: UCC28610 Green Mode Flyback Controller.
- SMPS Design and Topologies: Exploring different DC-DC converter topologies, including flyback and buck converters.
- Application of SMPS in Consumer Electronics: How SMPS are used in various consumer devices.
Note: You can find further design guides and application notes from Texas Instruments and other semiconductor manufacturers to assist in your SMPS design journey.